An early predictor of cognitive decline in Parkinson's disease
Have you ever felt the strong sensation that someone is behind you, so intense that you turn around, only to see that no-one is there? This is a 'presence hallucination’. Presence hallucinations are particularly frequent but underreported in patients with Parkinson’s disease and may appear early on in the course of the disease. They are sometimes ignored by the patient, by clinicians, or brushed off as a simple side-effect of medication.
Now, EPFL scientists have found that patients recently diagnosed with Parkinson’s disease and who have early hallucinations are at greater risk of faster cognitive decline. The results are published in Nature Mental Health.
“We now know that early hallucinations are to be taken seriously in Parkinson’s disease,” says Olaf Blanke, Bertarelli Chair in Cognitive Neuroprosthetics, who leads EPFL’s Laboratory of Cognitive Neuroscience.
“If you have Parkinson’s disease and experience hallucinations, even minor ones, then you should share this information with your doctor as soon as possible,” explains Fosco Bernasconi of EPFL’s Laboratory of Cognitive Neuroscience and lead author of the study. “So far, we only have evidence linking cognitive decline and early hallucinations for Parkinson’s disease, but it could also be valid for other neurodegenerative diseases.”
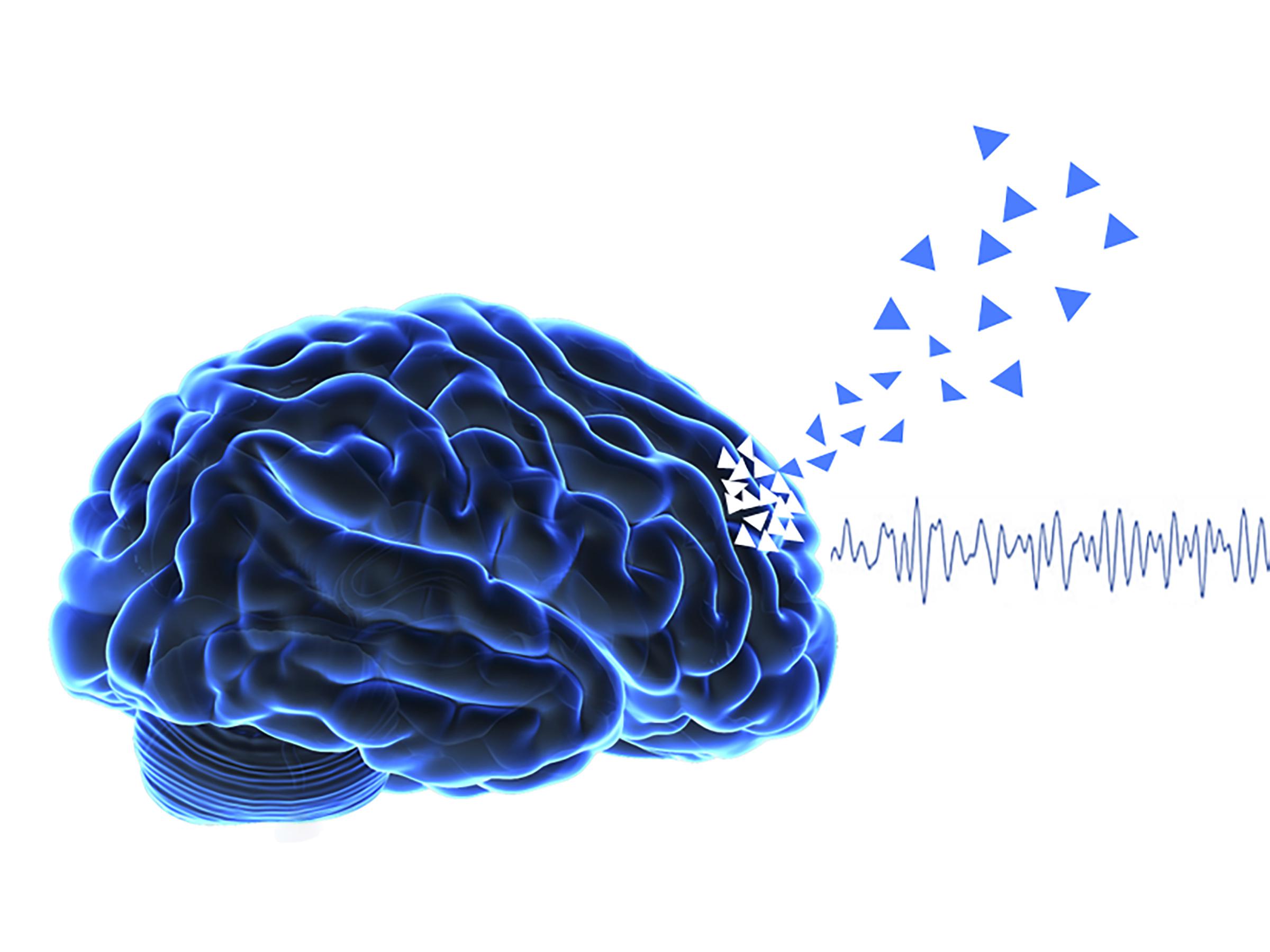
A long-term clinical study of Parkinson’s patients
In a collaboration between EPFL and Sant Pau Hospital in Barcelona, the scientists collected data about 75 patients between the ages of 60 and 70 and who were all diagnosed with Parkinson’s disease. The clinicians and scientists at Sant Paolo Hospital conducted a series of neuropsychological interviews to assess their cognitive status, neuropsychiatric interviews about whether or not they were experiencing hallucinations, and electroencephalography (EEG) measurements of the brain’s activity at rest.
In analyzing the data, the scientists found that in patients with Parkinson’s disease, the cognitive decline of frontal executive function is more rapid in the following 5 years for patients with early hallucinations. The level of cognitive decline over those 5 years is further associated with frontal theta (4-8Hz) oscillatory activity as measured by the EEG during the first visit, but only if you have hallucinations at the onset. For clinically and demographically similar patients, the only difference at the outset is that one group has early hallucinations and the other does not.
Early detection for early treatment
Neurodegenerative diseases like Parkinson’s are often detected when it’s too late, the disease too advanced, limiting the impact of preventative measures and disease-modifying therapies. Bernasconi, Blanke and their collaborators are aiming to change that, looking for early signs – like minor hallucinations – and ways to promote early intervention for slowing down progression of cognitive and psychiatric symptoms of the disease.
Hallucinations are among the lesser-known symptoms of Parkinson’s and are highly prevalent early on in the disease, with one individual out of two experiencing hallucinations regularly. Among the different hallucinations, early hallucinations are indeed a cause for concern since they appear in a third of Parkinson patients before onset of motor symptoms like trembling. Parkinson’s disease is traditionally defined as a movement disorder with the typical motor symptoms of resting tremor, rigidity, and bradykinesia, but it also leads to a wide variety of non-motor symptoms that appear early in the course of the disease.
Hallucinations can be described by a continuum of symptoms, from minor symptoms that usually occur early in the course of the disease like presence hallucinations, to more severe symptoms like visual hallucinations that appear later.
It has also already been established that complex visual hallucinations, like seeing someone who is not there, have been linked to cognitive decline and dementia in Parkinson’s disease and related neurodegenerative disorders like dementia with Lewy bodies. However, complex visual hallucinations usually occur at a later stage of the disease, limiting their use as an early marker for cognitive decline.
“Detecting the earliest signs of dementia means early management of the disease, allowing us to develop improved and personalized therapies that try to modify the course of the disease and improve cognitive function,” continues Blanke.
“We aim to have an early marker to identify individuals at risk of a more severe form of Parkinson’s disease, characterized by a more rapid cognitive decline and dementia, based on hallucinations proneness. And ideally identify those individuals even before hallucinations actually occur. We are therefore developing neurotechnology methods and procedures for that purpose,” says Bernasconi.